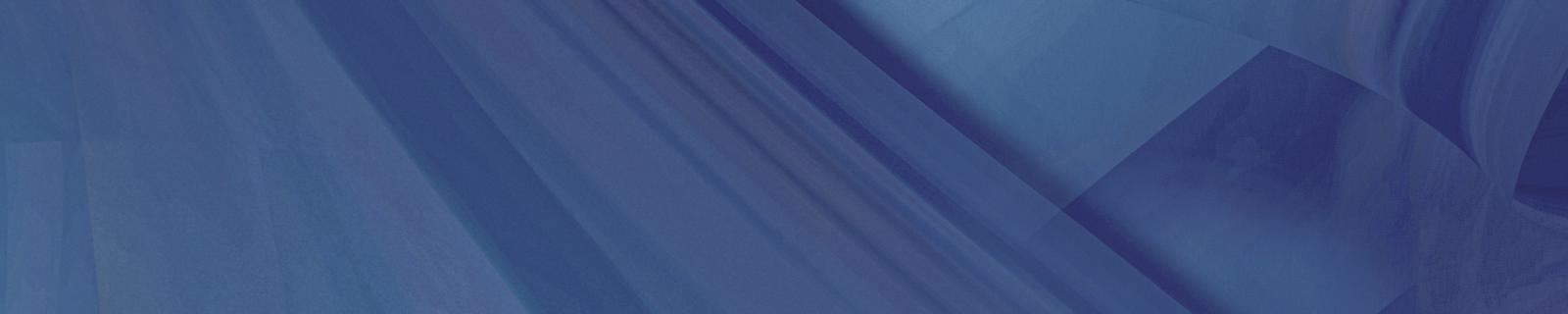
Neurophotonics Lab Research
We invent new technologies that use light to non-invasively “see” the structure and function of living biological tissues, deep below the surface. Often, these new imaging techniques are driven by fundamental insights into applied physics and engineering, which enable us to use light to form clearer pictures deep in turbid tissues or to measure physiology more accurately. Not satisfied with just a proof-of-concept, we strive to integrate these new technologies to drive basic scientific discovery and, in some cases, medical practice. We are housed in the Tech4Health Institute, which supports and enhances our laboratory’s mission.
Areas of Interest:
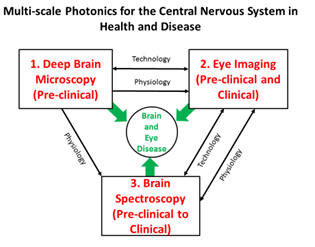
Representative Publications
1. Kholiqov O, Zhou W, Zhang T, Le VND, Srinivasan VJ. Time-of-Flight Resolved Light Field Fluctuations Reveal Deep Human Tissue Physiology. Nature Communications. 2020;11:391. DOI.
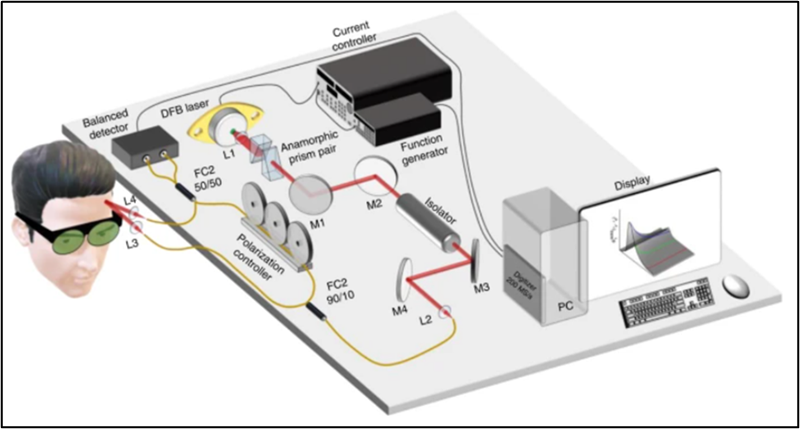
Results: We demonstrated a new technology to assess deep blood flow dynamics in living humans using near-infrared light. Using an optical “trick” of low coherence interferometry, the technique effectively gates multiply-scattered (diffuse) light from tissue with an unprecedented time-of-flight resolution of tens of picoseconds.
Impact: This work added two novel dimensions (optical phase and time-of-flight) to improve diffuse optics of blood flow. This work also showed the benefits of time-of-flight resolution for providing quantitative measurements, and more accurate blood flow measurements in layered media such as a human head.
2. Kho AM, Zhang T, Zhu J, Merkle CW, Srinivasan VJ. Incoherent Excess Noise Spectrally Encodes Broadband Light Sources. Light: Science & Applications. 2020;9:172. DOI.
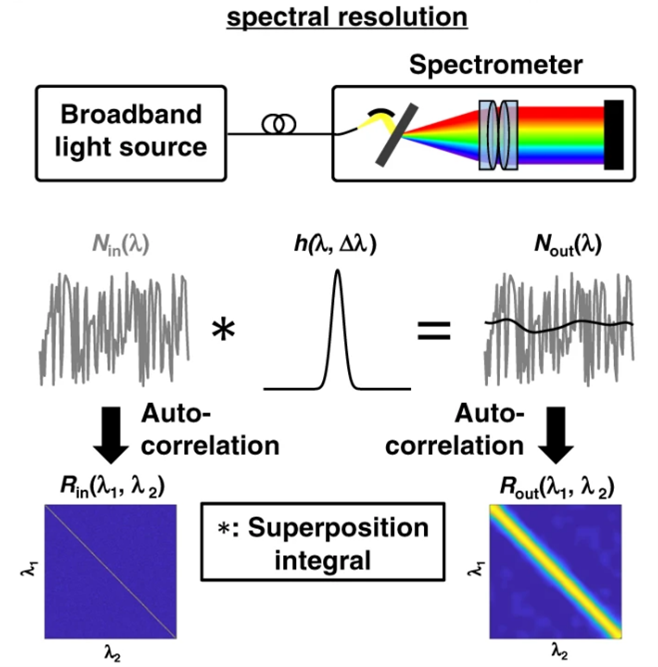
Results: Here, we showed that intrinsic noise fluctuations in two different kinds of light sources can serve a useful purpose: to characterize and cross-calibrate spectrometers. Using the characterization approach, we improved the spectrometer alignment of visible light optical coherence tomography, discovering a previously unseen band in the retina.
Impact: This work showed that excess noise, rather than being a nuisance as is often assumed, has many useful applications in optics and photonics. For instance, our cross-calibration approach can now align two different optical spectrometers to an extremely small fraction (1/100,000) of the overall spectral range, just by maximizing the noise correlations. This advance will enable highly precise and reproducible measurements of spectra, with applications in biomedical sciences, agriculture, and security.
3. Srinivasan VJ, Kho AM, Chauhan P. Visible Light Optical Coherence Tomography Reveals the Relationship of the Myoid and Ellipsoid to Band 2 in Humans. Transactions of Vision Science and Technology. 2022;11(9):3. DOI.
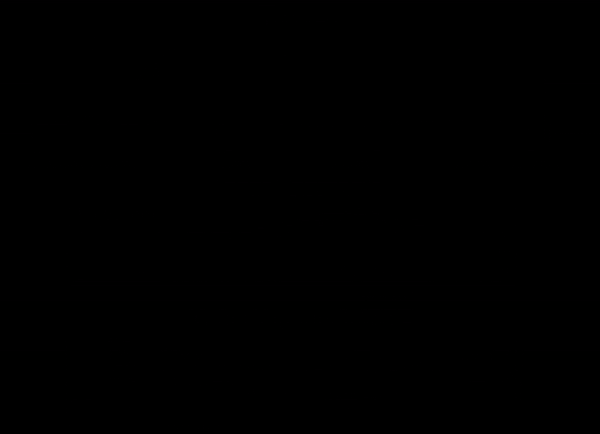
Segment 2: Visible light optical coherence tomography of the human fovea to near periphery
Segment 3: Visible light optical coherence tomography of the human perifovea to midperiphery
Results: We used a highly optimized visible light optical coherence tomography system with a world record 1.0 micron axial resolution to image the inner segments of the photoreceptors, showing an apparent division into an ‘m’ zone and an ‘e’ zone. The ‘m’ zone thickness paralleled known anatomy of the myoid, suggesting that the ‘e’ zone corresponds to the inner ellipsoid, which is rich in mitochondria.
Impact: This work shows that the inner ellipsoid is less scattering than the myoid, challenging the oft-cited assumption that all mitochondria in the retina are highly scattering. These data help to interpret outer retinal band 2, a clinically-important feature whose origins are controversial.
4. Zhou W, Kholiqov O, Zhu J, Zhao M, Zimmermann LL, Martin RM, Lyeth BG, Srinivasan VJ. Functional Interferometric Diffusing Wave Spectroscopy of the Human Brain. Science Advances. 2021;7(20). DOI.
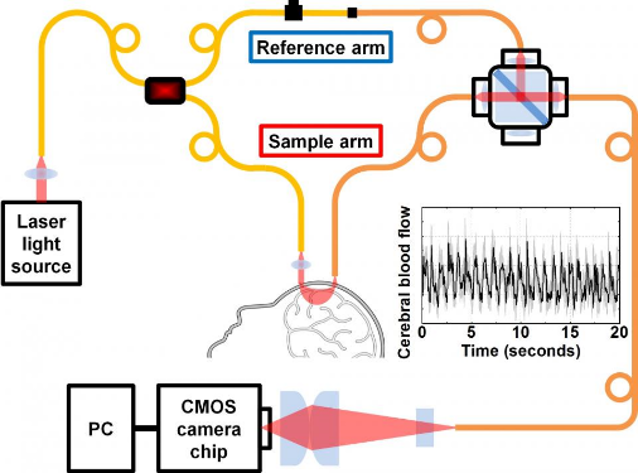
Results: In this work, using interferometry to provide optical gain, we transformed a CMOS camera into a sensitive device to measure weak light fluctuations from deep tissue. If well-matched with a multimode fiber, each pixel could perform comparably to, or better than, a single photon counting channel, and more robustly, at a fraction of the cost.
Impact: This work provided a fundamentally new approach to measuring brain blood flow, which will improve brain-computer interfaces and neurointensive care, and create a new class of wearable cerebral blood flow monitoring devices.
5. Zhu J, Freitas HR, Maezawa I, Jin LW, Srinivasan VJ. 1700 nm Optical Coherence Microscopy Enables Minimally Invasive, Label-Free, In Vivo Optical Biopsy Deep in the Mouse Brain. Light: Science & Applications. 2021;10:145. DOI.
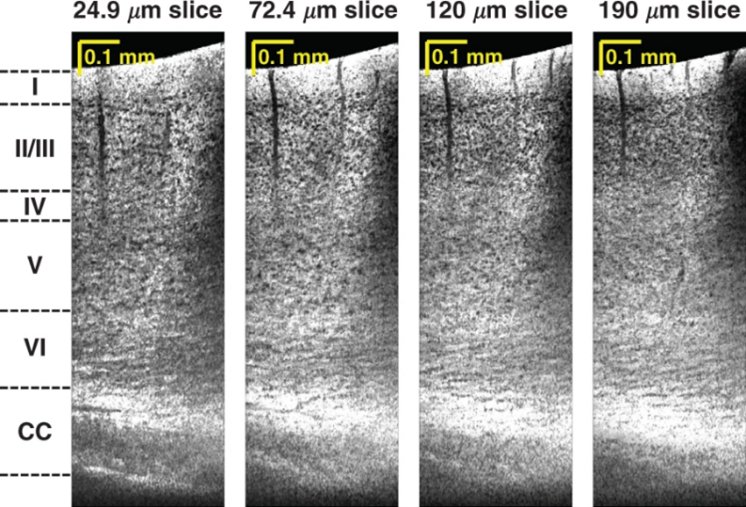
Results: This work achieved deep (~1.5 mm) mouse brain imaging through a minimally invasive thinned-skull preparation with optical coherence microscopy at 1700 nm. The utility of deep imaging was demonstrated in an Alzheimer’s disease model that differentially affects the deeper neocortical layers.
Impact: This work achieved a balance between deep and minimally invasive imaging of the mouse brain. Its unique combination of depth and minimal invasiveness compares favorably with even three-photon microscopy. The label-free images of the mouse brain approach the level of detail of histology, but are achieved in the living brain, without slicing or staining of the tissue. This line of research has prompted great interest in the use of the 1700 nm window for deep imaging, and we are working with several companies to address the technical challenges of this wavelength range.