Neuroscience Institute Research
Neuroscience Institute Journal Club
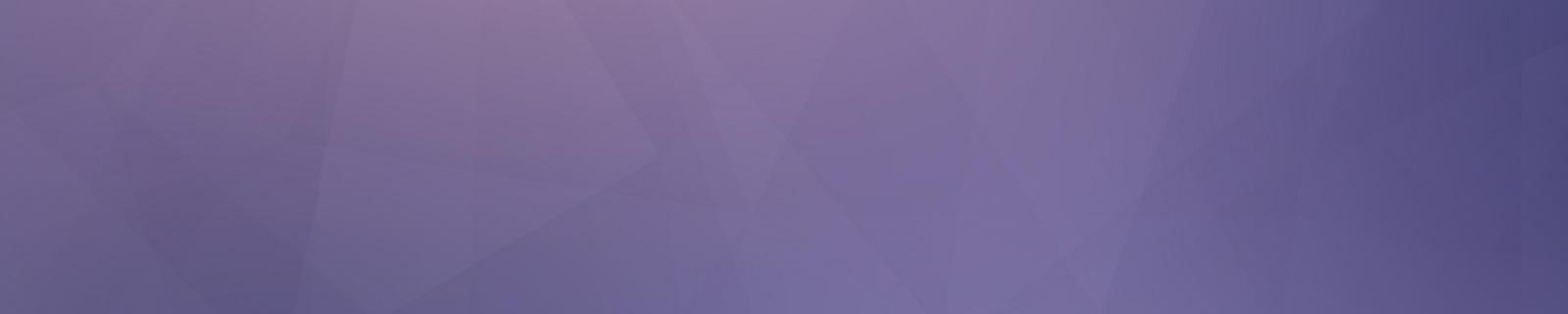
Neuroscience Institute Journal Club
The Neuroscience Institute Journal Club highlights high-profile work from our scientists-in-training, showcasing the quality, diversity, and innovation that characterize research at the Neuroscience Institute. We discuss the impact of our research on the field, on our understanding of the brain, and on potential breakthroughs that may translate to improved patient care.